A new Malaria vaccine – Can we finally conquer this challenging disease?
- tariromakadzange
- Sep 21, 2022
- 7 min read
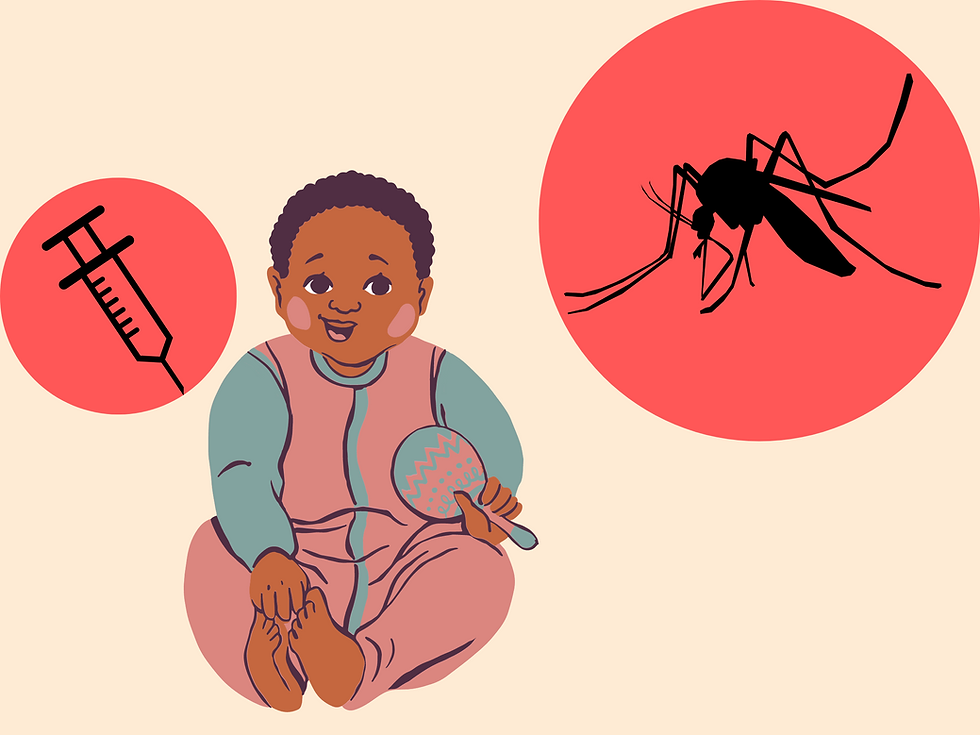
We recently heard the exciting news that a team from Oxford University had made significant advances in the development of a malaria vaccine. Data from their clinical trial showed that their vaccine continued to provide good protection against seasonal malaria in children following a booster dose. This is promising, particularly in a field where successful vaccine development has been challenging.
Malaria is caused by a parasite belonging to the plasmodium family and transmitted by the Anopheles mosquito. The anopheles mosquito family has over 500 species, 70 of which are vectors for malaria. The most effective and efficient dominant vector species within the Anopheles family are found in Africa. There are five major species of malaria however the largest burden of disease is caused by plasmodium falciparum. Although falciparum is distributed globally the afflicted areas are in sub-Saharan Africa, south-East Asia and the West Pacific. More than 2 billion people are at risk of falciparum malaria and each year there are over 200 million cases and 600,000 deaths. Most cases and deaths are in Africa.
Malaria is an ancient disease and cases have been well documented by early African, Chinese, European and Hindu civilizations. It was in the 1880s that much of the initial pathophysiology of the parasite was first understood, beginning when Charles Louis Alphonse Laveran, a French army officer working in Algeria discovered the parasites in blood samples in the 1880s. His observations opposed the prevailing wisdom that contended that the disease was caused by bacteria. His work was confirmed by other scientists who also began working on understanding the various stages of the parasite in the blood and the important role of the liver in the parasite’s development. Laveran went on to win the Nobel Prize in Medicine in 1907 for his work.
The complexity of the malaria life cycle has stimulated generations of malariologists but also taxed the memory of millions of students. Briefly, infection begins when sporozoites are injected by a mosquito into a human. The sporozoites make their way to liver cells where they undergo asexual reproduction leading to a form referred to as merozoites. These flood into the blood, invade red blood cells, and undergo another round of asexual replication. It is often during this stage that disease is observed. Some of the young merozoites develop into male and female gametocytes that circulate in the blood until they are taken up by a female Anopheles mosquito. At cooler temperatures than the human body, these gametocytes mature in the mosquito. Fertilization can occur with sexual reproduction that ultimately leads to the formation of sporozoites that migrate to the salivary gland of the mosquito. A mosquito bite to a human starts the cycle again.
Prior to understanding the role of the mosquito in transmission many believed that humans became infected by drinking contaminated water or by inhaling dust from dried up ponds where mosquitoes had died. It was not until the work of Ronald Ross working in India and Sierra Leone and studying similar parasites in birds that it became evident that the mosquito was an important part of the parasite lifecycle. This was confirmed by Italian scientists who conducted a voluntary exposure experiment that demonstrated that those who were protected from contact with mosquitoes did not acquire disease compared to those that were not. This became the basis of malaria prevention efforts that continue today including avoidance, screening, mosquito proofing of dwellings and draining mosquito habitats. Although these prevention measures are effective, they are often not sufficient, and there has been a decades long quest for the development of an effective vaccine.
In the 1970s researchers showed that radiation attenuated whole sporozoites induced protection from challenge with sporozoites but not blood stage parasites. Advances in molecular biology led to cloning of a major sporozoite surface antigen known as CSP. The protein was combined with T cell epitopes and attached to a hepatitis B surface antigen, resulting in the formation of highly immunogenic particles expressing the CSP fragment on their surface. This was combined with an adjuvant known as ASO1 resulting in the GSK developed Mosquirix vaccine. In phase 3 clinical trials the vaccine was given as 3 doses only or with a fourth booster dose. The vaccine reduced clinical episodes of malaria by 36% in young children and 26% in infants.
In 2015 Mosquirix (RTS,S/AS01E) received a favorable opinion from the European medicines authority following safety and efficacy data in young African children suggesting the use of the vaccine in children ages 6 weeks to 17 months. It was the first malaria vaccine to receive a favorable opinion from a major regulatory authority. However, efficacy waned over time. A study to address some questions that arose from the phase 3 study concerning cases of meningitis, cerebral malaria, gender specific outcomes and programmatic implementation was initiated in Malawi, Ghana, and Kenya. Initial data did not find any safety concerns for excess meningitis, cerebral malaria or gender specific mortality. Severe malaria was reduced by 30% and hospitalization by 21%. The study is expected to complete in June 2023 and ongoing data collection should provide sufficient power to evaluate impact on mortality.
Malaria is a seasonal disease and due to waning immunity with early vaccines many researchers have considered a seasonal boosting approach. A study looking at vaccine, chemoprophylaxis or both in Mali and Burkina Faso showed vaccination was non-inferior to chemoprophylaxis, but the combination was best in preventing uncomplicated, severe malaria and death. Until more efficacious vaccines are developed combination strategies will be necessary.
Further modifications in parasite antigen and delivery was carried out by a group at Oxford resulting in a vaccine that directly fuses CSP to hepatitis B surface antigen. In a Phase 1/2b study of children ages 5-17 months, the vaccine had an efficacy of up to 77% in the high adjuvant dose malaria vaccine group at 12-months follow up. Earlier this month the group reported data following a booster dose at 12 months which showed efficacy of 71% in the low adjuvant dose group and 80% in the high adjuvant dose group. This data is very encouraging as it shows that a booster dose has a role in maintaining efficacy. However, a larger phase 3 study is necessary in both areas of low and high transmission, with long term follow-up and evaluation of seasonal boosting. Notably, implementation studies suggest first booster drop-off in vaccination coverage rates can be as high as 30%. It will be important to understand the implementation challenges and to develop strategies for retention in a seasonal boosting strategy.
Continuous innovation and understanding of malaria immunobiology is enabling further advances in vaccine development. Although the Oxford data is encouraging, the primary antigen CSP has high sequence diversity. This means that efficacy may be limited to parasites that share similar sequences as the vaccine antigen and that escape variants may emerge. Monitoring this closely will be important for long term vaccine effectiveness. Secondly, a key fragment of the CSP has been demonstrated to induce protective immunity in children in Tanzania but is missing in both Mosquirix and the Oxford vaccine. Full length CSP vaccines are under development.
Recent advances in biology have allowed expansion of the early observations in the 1970s that showed that whole sporozoites induce good immune responses. Vaccines using attenuation methods such as radiation, chemo-attenuation and deletion of genes required to complete the liver stage development have been made. These vaccines have often been difficult to deliver because they require an ultra-cold chain, intravenous administration and further manufacturing optimization. However, in field trials in adults some of these vaccines have shown good efficacy. Unfortunately, some attenuation methods only work best in protecting against malaria parasites that are similar to the vaccine strain and do poorly against other variants.
Vaccines targeting the blood or disease stage of infection are an attractive target but have associated challenges due to the high degree of antigen variation in the blood stage parasites. To date vaccines targeting the blood stage parasites have been disappointing with low levels of efficacy. The next generation of blood stage vaccines in development are targeting antigens in highly conserved regions or proteins necessary for invasion of red blood cells.
Other targets for vaccine development are to block transmission by targeting the sexual stage of the parasite and blocking transmission to the mosquito. To date those vaccines have had minimal success with rapidly waning immunity.
Malaria and pregnancy can lead to poor maternal and fetal outcomes. It is estimated that over 30 million pregnancies a year in endemic countries are complicated by malaria infection. Prevention measures such as vector control and use of insecticide treated mosquito nets play an important role. However, a vaccine to protect against the complications of malaria in pregnancy is urgently needed. Placental targeting parasites express a distinctive protein that binds to receptors on the placenta. A few vaccines have been developed that target this protein. Some early data shows that these vaccines are safe and induce antibody responses. However the cross protection against parasites that differ from those included in the vaccine may not be as robust.
The WHO goal of achieving a vaccine with 75% efficacy by 2030 seems closer. Continued investments in understanding the immunobiology of the parasite and host interaction is critical for advancing the field and enabling the development of more efficacious vaccines. In addition to vaccine science including socio-behavioral and systems capacity research will be critical to enable effective implementation of a complex and possibly seasonal vaccination schedule. The routine immunization programs in Africa have not had to contend with seasonal vaccines and ensuring retention. Lastly it would also be critical for a malaria vaccine to be manufactured in Africa. Building the manufacturing capabilities for vaccines such as the Oxford vaccine on the continent would be beneficial and a test case for establishing the vaccine manufacturing ecosystem that is needed to sustain vaccine manufacturing on the continent.
*A. Tariro Makadzange is the author of this blog. She is an infectious disease physician and viral immunologist focused on tackling healthcare programs in Africa.
*Ruvimbo Chigumbu contributed research assistance.
*Nyasha Elose contributed editorial assistance.
Comments